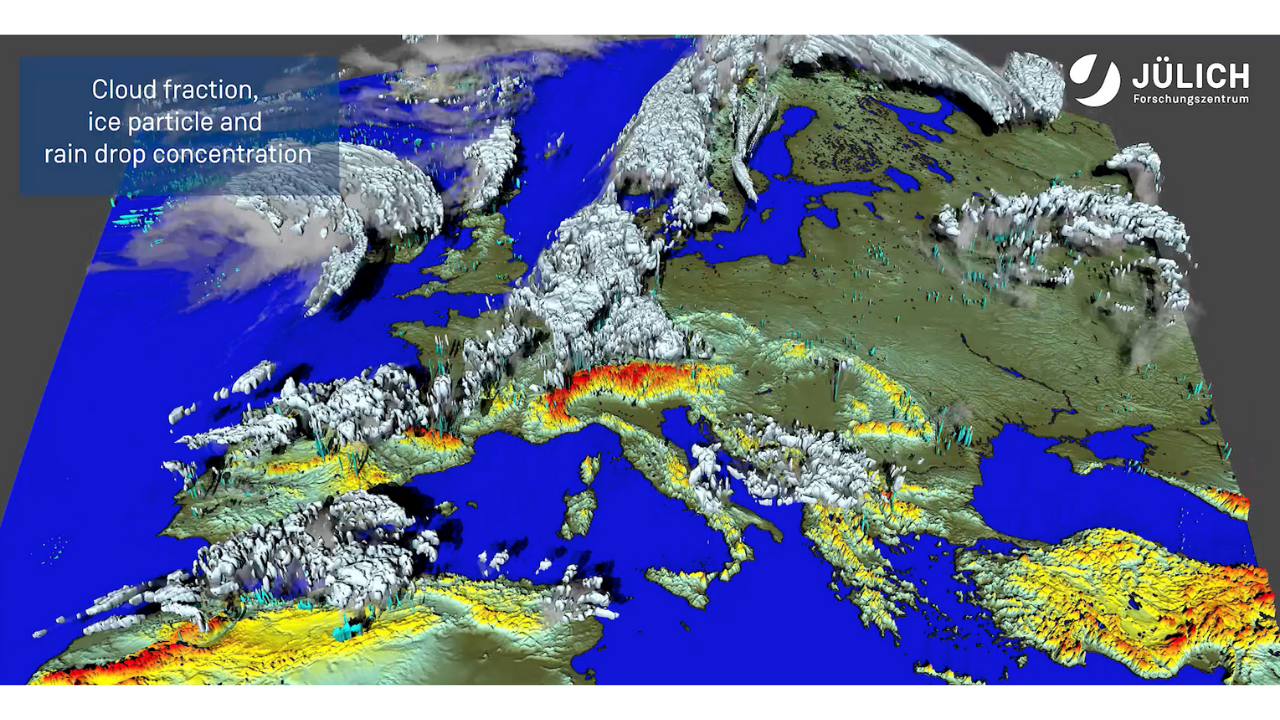
Written by Klaus Goergen from the Institute of Bio- and Geosciences, Agrosphere (IBG-3), Research Centre Jülich (FZJ), 52425 Jülich, Germany, and the Centre for High-Performance Scientific Computing in Terrestrial Systems (HPSC-TerrSys), Geoverbund ABC/J, 52428 Jülich, Germany. Contact: k.goergen@fz-juelich.de.
Regional climate model (RCM) simulations are often used to dynamically downscale global climate models (GCMs), i.e., the initial conditions, lateral boundary conditions, and also the lower boundary conditions over the oceans in case of an uncoupled model, are obtained from the GCM (Rockel, 2015). In such a dynamically downscaling setup, where the RCMs are run over a spatially limited area of the Earth at higher resolution, they can add value to the GCMs at coarser spatial resolution, because small-scale surface heterogeneities can be better represented due to orography, coastlines, lakes, landcover, and mesoscale dynamical processes as well as extreme events (Rummukainen, 2016). Typical RCM spatial resolutions nowadays range between 12km and 25km, e.g., in the Coordinated Regional Downscaling Experiment (CORDEX) (Jacob et al., 2020; Gutowski et al., 2016), alongside GCMs, that feature horizontal resolutions of typically around 100km or above (Eyring et al., 2016), whereas higher-resolution CMIP6 experiments do exist (Haarsma et al., 2016). CORDEX and CMIP experiments are part of the World Climate Research Programme (WCRP), and results from these simulations are currently used in the 6th Assessment Report (6AR) of the Intergovernmental Panel on Climate Change (IPCC) (IPCC, 2021).
Since first simulations in the 1990s, RCMs have undergone a long development history and advancements (Dickinson et al., 1989; Giorgi, 1990; Giorgi, 2019; Rockel, 2015). They are used in a wide range of applications, e.g., as part of regional climate change simulations, and are often organized as community multi-model ensemble experiments, such as CORDEX, that constitute the basis of many climate change impact or adaptation studies (Jacob et al., 2020; Gutowski et al., 2016; Gutowski et al., 2020). An important development in recent years are RCM simulations at convection-permitting resolution, where error-prone deep convection parametrizations can be switched off. The benefits as well as the challenges of such high-resolution km-scale (usually below 4km horizontal resolution) simulations are manifold, see summaries, e.g., in Prein et al. (2015) and Schär et al. (2020). Computational challenges are, for example, related to the small timesteps that are needed for a numerically stable integration of the non-hydrostatic governing equations, and the very large data volumes due to short output frequencies, higher lateral boundary input frequencies, or the higher resolution vertical discretisation, to name a few (Prein et al., 2015).
As convection-permitting regional climate modelling can contribute to specific CORDEX scientific challenges, one of the first CORDEX Flagship Pilot Studies (FPS) has been the FPS “Convective phenomena at high resolution over Europe and the Mediterranean” (FPS-CONV) in 2016. Objectives of FPS-CONV are to investigate convective-scale events, processes, and changes, assess modelling capacities at km-scale resolution, and to investigate how climate change alters the impacts of convective events. Such events are of particular importance as they often lead to damaging weather events, with heavy rainfall, consecutive flash floods, short-lived windstorms, hail and/or lightning (e.g., Ducrocq et al. 2014).
Based on dedicated compute time projects on PPI4HPC supported infrastructures at the Jülich Supercomputing Centre (JSC), the JURECA cluster module and JURECA-DC module, FZJ staff together with collaborators have contributed – aside from individual studies – to the joint coordinated multi-model, multi-physics ensemble experiments and analyses of the FPS-CONV. Here, currently nine different RCMs and 29 modelling groups from all over Europe collaborate along three large community experiments, that are central to the FPS-CONV, which are described in the following:
Initial implementation and km-scale RCM functionality tests, as documented in (Coppola et al., 2020), cover three extreme precipitation events. For these short event simulations, the RCMs can already show, based on different initialisations, a higher level of realism in reproducing the spatial distribution, the diurnal cycle, and the intensities of precipitation. Events strongly driven by large scale weather systems exhibit, as expected, higher correlation skill scores. Also, local-scale interactions between the flow and orography and land-ocean contrasts become apparent. An RCM sub-group using the WRF model, whose initial km-scale model configuration was based simulations by Knist et al. (2020) on the JURECA HPC system, allows for a constrained and coordinated multi-physics experiment design for a separation of cause-and-effect relationships (e.g., Lavin-Gullon et al., 2021).
In an ensuing experiment, a 10-year evaluation run 22-member RCM ensemble (driven by ERA-interim reanalysis) for a convection-permitting greater Alpine region model domain is analysed (Ban et al., 2021). Comparing mean precipitation, precipitation intensity and frequency, and heavy precipitation on daily and hourly timescales from the km-scale and lower resolution (driving models, around 10-15km) RCMs and observations in different seasons, shows a more realistic precipitation simulation in the km-scale RCMs. This is especially true for heavy precipitation and precipitation frequencies on both daily and hourly time scales during the summer season. This is a demonstration for a clear added value of the convection-permitting RCM runs, that justifies the manifold computational effort, just in terms of number of iterations.
The impact of climate change is investigated in a companion 10-year multi-model ensemble study, where selected CMIP5 GCM simulations over historical and end-of-century time slices are jointly analysed (Pichelli et al., 2021). Like the simulations above, this is a first-of-its-kind km-scale study of projected precipitation changes over the greater Alpine domain within a coordinated study such as the CORDEX FPS-CONV. An ensemble of twelve simulations is analysed. The km-scale climate change ensemble is, as above, the apparent added value. Apart from a refinement and an enhancement of projected changes with reference to coarser resolution RCMs, the km-scale model runs show larger amplitudes of change and larger positive changes of daily and hourly precipitation extremes. Generally, the changes are indicative of an intensified future regional and local hydrological cycle.
The JSC JURECA cluster module and JURECA-DC module are especially well suited for these kinds of experiments that are run in distributed or hybrid memory parallelism in a strong-scaling setup, where the fixed model domain size limits scalability to a few nodes only (e.g., three nodes on JURECA-DC utilising 384 cores gives a good parallel efficiency level), before communication overhead with the 2D (pencil) decomposition becomes too large. The output of these simulations per month ranges between 200 and 500GB using parallel I/O to shared netCDF files. A substantial data volume reduction goes along the transfer of data to CMOR standard, as a prerequisite for staging data on Earth System Grid Federation (ESGF) data nodes to meet FAIR standards.
Current developments are towards larger convection-permitting model domains (e.g., Berthou et al., 2018) and longer time-slices, also using fully coupled model systems (e.g., Gasper et al., 2014; Shrestha et al., 2014), which feature, e.g., a sophisticated groundwater representation (Kuffour et al., 2020) that allows to access water resources questions in combination with the added value from km-scale RCMs.
References:
Ban, N., Caillaud, C., Coppola, E., Pichelli, E., Sobolowski, S., Adinolfi, M., et al. (2021). The first multi-model ensemble of regional climate simulations at kilometer-scale resolution, part I: evaluation of precipitation. Climate Dynamics. https://doi.org/10.1007/s00382-021-05708-w
Berthou, S., Kendon, E. J., Chan, S. C., Ban, N., Leutwyler, D., Schr, C., & Fosser, G. (2018). Pan-European climate at convection-permitting scale: a model intercomparison study. Climate Dynamics. https://doi.org/10.1007/s00382-018-4114-6
Coppola, E., Sobolowski, S., Pichelli, E., Raffaele, F., Ahrens, B., Anders, I., et al. (2020). A first-of-its-kind multi-model convection permitting ensemble for investigating convective phenomena over Europe and the Mediterranean. Climate Dynamics, 55(1-2), 3-34. https://doi.org/10.1007/s00382-018-4521-8
Dickinson, R. E., Errico, R. M., Giorgi, F., & Bates, G. T. (1989). A regional climate model for the western United States. Climatic Change, 15(3), 383-422. https://doi.org/10.1007/BF00240465
Ducrocq, V., Braud, I., Davolio, S., Ferretti, R., Flamant, C., Jansa, A., et al. (2014). HyMeX-SOP1: The Field Campaign Dedicated to Heavy Precipitation and Flash Flooding in the Northwestern Mediterranean. Bulletin of the American Meteorological Society, 95(7), 1083-1100. https://journals.ametsoc.org/view/journals/bams/95/7/bams-d-12-00244.1.xml
Eyring, V., Bony, S., Meehl, G. A., Senior, C. A., Stevens, B., Stouffer, R. J., & Taylor, K. E. (2016). Overview of the Coupled Model Intercomparison Project Phase 6 (CMIP6) experimental design and organization. Geoscientific Model Development, 9(5), 1937-1958. http://www.geosci-model-dev.net/9/1937/2016/
Gasper, F., Goergen, K., Shrestha, P., Sulis, M., Rihani, J., Geimer, M., & Kollet, S. (2014). Implementation and scaling of the fully coupled Terrestrial Systems Modeling Platform (TerrSysMP v1.0) in a massively parallel supercomputing environment – a case study on JUQUEEN (IBM Blue Gene/Q). Geoscientific Model Development, 7(5), 2531-2543. http://doi.org/10.5194/gmd-7-2531-2014
Giorgi, F. (1990). Simulation of regional climate using a limited area model nested in a general circulation model. Journal of Climate, 3(9), 941-963. https://doi.org/10.1175/1520-0442(1990)003%3C0941:SORCUA%3E2.0.CO;2
Giorgi, F. (2019). Thirty Years of Regional Climate Modeling: Where Are We and Where Are We Going next? Journal of Geophysical Research: Atmospheres, 124(11), 5696-5723. https://agupubs.onlinelibrary.wiley.com/doi/abs/10.1029/2018JD030094
Gutowski Jr, W. J., Giorgi, F., Timbal, B., Frigon, A., Jacob, D., Kang, H. S., et al. (2016). WCRP COordinated Regional Downscaling EXperiment (CORDEX): a diagnostic MIP for CMIP6. Geoscientific Model Development, 9(11), 4087-4095. https://gmd.copernicus.org/articles/9/4087/2016/
Gutowski, W. J., Jr., Ullrich, P. A., Hall, A., Leung, L. R., O’Brien, T. A., Patricola, C. M., et al. (2020). The Ongoing Need for High-Resolution Regional Climate Models: Process Understanding and Stakeholder Information. Bulletin of the American Meteorological Society, 101(5), E664-E683. https://doi.org/10.1175/BAMS-D-19-0113.1
IPCC, 2021: Climate Change 2021: The Physical Science Basis. Contribution of Working Group I to the Sixth Assessment Report of the Intergovernmental Panel on Climate Change [Masson-Delmotte, V., P. Zhai, A. Pirani, S. L. Connors, C. Péan, S. Berger et al. (eds.)]. Cambridge University Press. In Press.
Jacob, D., Teichmann, C., Sobolowski, S., Katragkou, E., Anders, I., Belda, M., et al. (2020). Regional climate downscaling over Europe: perspectives from the EURO-CORDEX community. Regional Environmental Change, 20(2), 51. https://doi.org/10.1007/s10113-020-01606-9
Knist, S., Goergen, K., & Simmer, C. (2020). Evaluation and projected changes of precipitation statistics in convection-permitting WRF climate simulations over Central Europe. Climate Dynamics, 55(1-2), 325-341. https://dx.doi.org/10.1007/s00382-018-4147-x
Kuffour, B. N. O., Engdahl, N. B., Woodward, C. S., Condon, L. E., Kollet, S., & Maxwell, R. M. (2020). Simulating coupled surface–subsurface flows with ParFlow v3.5.0: capabilities, applications, and ongoing development of an open-source, massively parallel, integrated hydrologic model. Geosci. Model Dev., 13(3), 1373-1397. https://gmd.copernicus.org/articles/13/1373/2020/
Lavin-Gullon, A., Fernandez, J., Bastin, S., Cardoso, R. M., Fita, L., Giannaros, T. M., et al. (2021). Internal variability versus multi-physics uncertainty in a regional climate model. International Journal of Climatology, 41, E656–E671. https://rmets.onlinelibrary.wiley.com/doi/abs/10.1002/joc.6717
Pichelli, E., Coppola, E., Sobolowski, S., Ban, N., Giorgi, F., Stocchi, P., et al. (2021). The first multi-model ensemble of regional climate simulations at kilometer-scale resolution part 2: historical and future simulations of precipitation. Climate Dynamics, 56(11), 3581-3602. https://doi.org/10.1007/s00382-021-05657-4
Prein, A. F., Langhans, W., Fosser, G., Ferrone, A., Ban, N., Goergen, K., et al. (2015). A review on regional convection-permitting climate modeling: Demonstrations, prospects, and challenges. Reviews of Geophysics, 53(2), 323-361. http://doi.org/10.1002/2014RG000475
Rockel, B. (2015). The Regional Downscaling Approach: a Brief History and Recent Advances. Current Climate Change Reports, 1(1), 22-29. https://doi.org/10.1007/s40641-014-0001-3
Rummukainen, M. (2016). Added value in regional climate modeling. Wiley Interdisciplinary Reviews: Climate Change, 7(1), 145-159. http://doi.wiley.com/10.1002/wcc.378
Shrestha, P., Sulis, M., Masbou, M., Kollet, S., & Simmer, C. (2014). A scale-consistent Terrestrial Systems Modeling Platform based on COSMO, CLM, and ParFlow. Monthly Weather Review, 142(9), 3466-3483. http://journals.ametsoc.org/doi/abs/10.1175/MWR-D-14-00029.1
Schär, C., Fuhrer, O., Arteaga, A., Ban, N., Charpilloz, C., Di Girolamo, S., et al. (2020). Kilometer-scale climate models: Prospects and challenges. Bulletin of the American Meteorological Society, 101(5), E567–-E587. https://doi.org/10.1175/BAMS-D-18-0167.1